图书介绍
Understanding enzymes: functionPDF|Epub|txt|kindle电子书版本下载
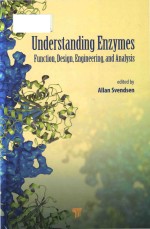
- 著
- 出版社: Pan Stanford Publishing Pte Ltd
- ISBN:
- 出版时间:2016
- 标注页数:0页
- 文件大小:309MB
- 文件页数:914页
- 主题词:
PDF下载
下载说明
Understanding enzymes: functionPDF格式电子书版下载
下载的文件为RAR压缩包。需要使用解压软件进行解压得到PDF格式图书。建议使用BT下载工具Free Download Manager进行下载,简称FDM(免费,没有广告,支持多平台)。本站资源全部打包为BT种子。所以需要使用专业的BT下载软件进行下载。如BitComet qBittorrent uTorrent等BT下载工具。迅雷目前由于本站不是热门资源。不推荐使用!后期资源热门了。安装了迅雷也可以迅雷进行下载!
(文件页数 要大于 标注页数,上中下等多册电子书除外)
注意:本站所有压缩包均有解压码: 点击下载压缩包解压工具
图书目录
PART Ⅰ ENZYME FUNCTION3
1 A Short Practical Guide to the Quantitative Analysis of Engineered Enzymes&Christopher D.Bayer and Florian Hollfelder3
1.1 Introduction3
1.2 Quantifying Reaction Progress4
1.3 Typical Saturation Plots Give Michaelis-Menten Parameters5
1.4 What Can Go Wrong?8
1.5 Dealing with Multiphasic and Pre-Steady-State Kinetics12
1.6 Evaluating Enzymes16
2 Protein Conformational Motions:Enzyme Catalysis&Xinyi Huang,C.Tony Liu,and Stephen J.Benkovic21
2.1 Introduction21
2.2 Multidimensional Protein Landscape and the Timescales of Motions22
2.3 Conformational Changes in Enzyme-Substrate Interactions26
2.4 Conformational Changes in Catalysis28
2.4.1 Protein Dynamics of DHFR in the Catalytic Cycle30
2.4.2 Temporally Overlap:Correlation Does Not Mean Causation32
2.4.3 Fast Timescale Conformational Fluctuations34
2.4.4 Effect of Conformational Changes on the Electrostatic Environment36
2.5 Conservation of Protein Motions in Evolution38
2.6 Designing Protein Dynamics39
2.7 Concluding Remarks40
3 Enzymology Meets Nanotechnology:Single-Molecule Methods for Observing Enzyme Kinetics in Real Time&Kerstin G.Blank,Anna A.Wasiel,and Alan E.Rowan47
3.1 Introduction48
3.2 Single-Turnover Detection53
3.2.1 Fluorescent Reporter Systems53
3.2.2 Measurement Setup56
3.2.3 Data Analysis57
3.3 Single-Enzyme Kinetics60
3.3.1 Candida antarctica Lipase B63
3.3.2 Thermomyces lanuginosus Lipase67
3.3.3 α-Chymotrypsin73
3.3.4 Nitrite Reductase78
3.3.5 Summary84
3.4 New Developments Facilitated by Nanotechnology88
3.4.1 Nano-optical Approaches89
3.4.2 Nano-electronic Approaches96
3.4.3 Nanomechanical Approaches103
3.4.4 Summary108
3.5 Conclusion110
4 Interfacial Enzyme Function Visualized Using Neutron,X-Ray,and Light-Scattering Methods&Hanna Wacklin and Tommy Nylander125
4.1 Phospholipase A2:An Interfacially Activated Enzyme126
4.1.1 Neutron Reflection129
4.1.2 Ellipsometry130
4.1.3 Activity of Naja mossambica mossambica PLA2130
4.1.4 Fate of the Reaction Products133
4.1.5 The Lag Phase and Activation of Pancreatic PLA2135
4.1.6 Distribution of Products during the Lag Phase138
4.1.7 Hydrolysis of DPPC by Pancreatic PLA2139
4.1.8 Role of the Reaction Products in PLA2 Activation141
4.1.9 Effect of pH and Activation by Me-β-cyclodextrin144
4.2 Other Lipolytic Enzyme Reactions on Surfaces150
4.2.1 Triacylglycerol Lipases and the Role of Lipid Liquid Crystalline Nanostructures150
4.3 Cellulase Enzymes154
4.4 Conclusion158
5 Folding Dynamics and Structural Basis of the Enzyme Mechanism of Ubiquitin C-Terminal Hydroylases&Shang-Te Danny Hsu167
5.1 Introduction169
5.1.1 UCH-L1171
5.1.1.1 Genetic association between UCH-L1 and neurodegenerative diseases171
5.1.1.2 UCH-L1 in oncogenesis175
5.1.2 Molecular Insights into the Pathogenesis Associated with UCH-L1175
5.1.3 UCHL3177
5.1.4 UCHL5178
5.1.5 BAP1179
5.2 UCH Structures180
5.3 Folding Dynamics and Kinetics183
5.4 Substrate Recognition184
5.5 Enzyme Mechanism186
5.6 Conclusion189
6 Stabilization of Enzymes by Metal Binding:Structures of Two Alkalophilic Bacillus Subtilases and Analysis of the Second Metal-Binding Site of the Subtilase Family&Jan Dohnalek,Katherine E.McAuley,Andrzej M.Brzozowski,Peter R.φstergaard,Allan Svendsen,and Keith S.Wilson203
6.1 Introduction:Subtilases and Metal Binding203
6.1.1 Calcium-Binding Sites in Bacillus:Proposal for a Standard Nomenclature209
6.1.2 The Weak Metal-Binding Site214
6.2 Two New Structures of Subtilases with Altered Calcium Sites216
6.2.1 Proteinase SubTY216
6.2.1.1 The overall fold216
6.2.1.2 The active site216
6.2.1.3 SubTY calcium and sodium sites218
6.2.1.4 SubTY disulfide bridge219
6.2.2 SubHal220
6.2.2.1 The unliganded form of SubHal220
6.2.2.2 The SubHal:CI2A complex221
6.2.2.3 Termini,surface,and pH stability of SubHal221
6.2.2.4 The two crystallographically independent SubHal:CI2A complexes223
6.2.2.5 The calcium sites in SubHal224
6.2.2.6 The active site of SubHal226
6.2.3 Enzymatic Activity of SubTY and SubHal228
6.2.4 Comparison of SubTY and SubHal with Other Subtilases228
6.2.5 The SubHal C-domain Compared to the Eukaryotic PCs,Furin and Kexin232
6.2.5.1 Active site comparison233
6.2.5.2 The specificity pockets234
6.2.5.3 Inhibitor CI2A binding234
6.2.6 Activity Profiles236
6.2.7 Comparison of Metal Binding at the Strong and Weak Sites in the S8 Family236
6.2.8 The Ca-Ⅱ and Na-Ⅱ Metal-Binding Sites237
6.3 Conclusion:Implications for Structural Studies of Enzymes248
6.4 Materials and Methods249
6.4.1 SubTY249
6.4.1.1 Protein production and purification249
6.4.1.2 Purification of the SubTY:CI2A (1:1)complex250
6.4.1.3 Crystallization250
6.4.1.4 Structure determination 2516.4.2 SubHal251
6.4.2.1 Protein production and purification251
6.4.2.2 Purification of the SubHal:CI2A (1:1)complex252
6.4.2.3 Crystallization252
6.4.2.4 Structure determination253
6.4.3 Protease Assays256
6.4.4 pH Stability257
6.4.5 Data Deposition257
7 Structure and Functional Roles of Surface Binding Sites in Amylolytic Enzymes&Darrell Cockburn and Birte Svensson267
7.1 Introduction267
7.2 Identifiication of SBSs:X-Ray Crystallography271
7.3 Bioinformatics of SBS Enzymes273
7.4 Binding Site Isolation275
7.5 Protection of Binding Sites from Chemical Labeling277
7.6 Nuclear Magnetic Resonance277
7.7 Binding Assays278
7.8 Activity Assays282
7.9 Future Prospects283
7.10 Conclusion286
8 Interfacial Enzymes and Their Interactions with Surfaces:Molecular Simulation Studies&Nathalie Willems,Mickael Lelimousin,Heidi Koldsφ,and Mark S.P.Sansom297
8.1 Introduction297
8.2 Enzyme Interactions at Interfaces299
8.3 Molecular Dynamic Simulations of Biomolecular Systems301
8.4 Lipases303
8.4.1 Atomistic MD Studies of Lipase Interactions with Interfaces304
8.4.2 The Role of Water in Lipase Catalysis at Interfaces307
8.5 Coarse-Grained MD Studies of Interfacial Enzymes:Orientation and Interactions309
8.5.1 Phospholipase A2309
8.5.2 PTEN310
8.6 Conclusions311
PART Ⅱ ENZYME DESIGN321
9 Sequence,Structure,Function:What We Learn from Analyzing Protein Families&Michael Widmann and Jurgen Pleiss321
9.1 Introduction321
9.2 Detection of Inconsistencies Utilizing a Standard Numbering Scheme323
9.3 Identification of Functionally Relevant Positions327
9.4 The Modular Structure of Thiamine Diphosphate-Dependent Decarboxylases330
9.5 Stereoselectivity-Determining Positions:The S-Pocket Concept in Thiamine Diphosphate-Dependent Decarboxylases333
9.6 Regioselectivity-Determining Positions:Design of Smart Cytochrome P450 Monooxygenase Libraries336
9.7 Substrate Specificity-Determining Positions:The GX/GGGX Motif in Lipases340
9.8 Conclusion341
10 Bioinformatic Analysis of Protein Families to Select Function-Related Variable Positions&Dmitry Suplatov,Evgeny Kirilin,and Vytas Svedas351
10.1 Introduction352
10.2 Bioinformatic Analysis of Evolutionary Information to Identify Function-Related Variable Positions359
10.2.1 Problem Definition359
10.2.2 Scoring Schemes in the Variable Position Selection:High-Entropy,Subfamily-Specific,and Co-Evolving Positions361
10.2.3 Association of the Variable Positions with Functional Subfamilies366
10.2.4 How to Select Functionally Important Positions as Hotspots for Further Evaluation:Implementation of Statistical Analysis366
10.3 The Bioinformatic Analysis of Diverse Protein Superfamilies369
10.3.1 Bioinformatic Challenges at Studying Enzymes369
10.3.2 Zebra:A New Algorithm to Select Functionally Important Subfamily-Specific Positions from Sequence and Structural Data370
10.4 Subfamily-Specific Positions as a Tool for Enzyme Engineering375
10.5 Conclusion377
11 Decoding Life Secrets in Sequences by Chemicals&Zizhang Zhang387
11.1 Introduction388
11.2 Linking an Enzyme’s Activity to Its Sequence389
11.3 Refiining the Sequence Space to a Specifiic Function by Directed Evolution395
11.4 Linking Chemistry to -Omics with High-Throughput Screening Methods398
11.5 Finding Large Sequence Space of a Specific Function from Microbial Diversity400
11.6 Linking Sequences to Substromes at the Molecular Level404
11.6.1 Biocatalytic Study of EHs405
11.6.2 Pharmacological Study of EHs407
11.6.3 Mechanistic Study of EHs407
11.6.4 What We Have Learned from the Studies of EH410
11.6.5 Technologies with Potentials in Genochemistry Approach410
11.7 Correlating with Computational Methods410
11.8 Problems That Genochemistry Can Potentially Tackle413
11.9 Conclusion 41412 Role of Tunnels and Gates in Enzymatic Catalysis&Sergio M.Marques,Jan Brezovsky,and Jiri Damborsky421
12.1 Introduction421
12.2 Protein Tunnels423
12.2.1 Structural Basis and Function423
12.2.2 Identification Methods427
12.2.3 Molecular Engineering429
12.3 Protein Gates431
12.3.1 Structural Basis and Function431
12.3.2 Identification Methods437
12.3.3 Molecular Engineering440
12.4 Conclusions442
13 Molecular Descriptors for the Structural Analysis of Enzyme Active Sites&Valerio Ferrario,Lydia Siragusa,Cynthia Ebert,Gabriele Cruciani,and Lucia Gardossia465
13.1 Introduction:Molecular Descriptors for Investigation of Enzyme Catalysis465
13.2 Molecular Descriptors Based on Molecular Interaction Fields467
13.3 Multivariate Statistical Analysis for Processing and Interpretation of Molecular Descriptors472
13.4 Grind Descriptors for the Study of Substrate Specificity475
13.5 VolSurf Descriptors for the Modeling of Substrate Specifiicity477
13.6 Differential MIFs Descriptors for the Study of Enantioselectivity479
13.7 Hybrid MIFs Descriptors for the Computation of Entropic Contribution to Enantiodiscrimination481
13.8 Analysis of Enzyme Active Sites for Rational Enzyme Engineering484
13.9 BioGPS Descriptors for in silico Rational Design and Screening of Enzymes489
13.10 Conclusions495
14 Hydration Effects on Enzyme Properties in Nonaqueous Media Analyzed by MD Simulations&Diana Lousa,Antonio M.Baptista,and Claudio M.Soares501
14.1 Enzyme Reactions in Nonaqueous Solvents502
14.2 Classes of Nonaqueous Solvents503
14.3 The Role of Water in Nonaqueous Biocatalysis504
14.4 Effect of Water Content on Enzyme Structure and Dynamics504
14.5 Effect of Water Content on Enzyme Selectivity507
14.6 Hydration Mechanisms of Enzymes in Polar and Nonpolar Solvents508
14.7 Enzyme Behavior as a Function of Water Activity510
14.8 Hydration Effects on Enzyme Reactions in Ionic Liquids512
14.9 Hydration Effects on Enzyme Reactions in Supercritical Fluids514
14.10 Conclusions516
15 Understanding Esterase and Amidase Reaction Specificities by Molecular Modeling&Per-Olof Syren523
15.1 Introduction523
15.2 Fundamental Catalytic Concepts525
15.2.1 Fundamental Chemistry of Amides and Esters525
15.2.2 Esterases and Amidases and Their Metabolic Significance525
15.2.3 Fundamental Chemical Aspects of Amidase and Esterase Catalysis526
15.2.4 Impact of Stereoelectronic Effects on the Enzymatic Reaction Mechanism529
15.3 Molecular Modeling of Fundamental Catalytic Concepts529
15.3.1 QM Calculations on Amidases and Esterases529
15.3.2 MD Simulations on Amidases and Esterases535
15.3.3 QM/MM Simulations on Amidases and Esterases539
15.4 Outlook and Implications for Enzyme Design544
15.5 Additional Comments546
PART Ⅲ ENZYME DIVERSITY561
16 Toward New Nonnatural TIM-Barrel Enzymes Using Computational Design and Directed Evolution Approaches&Mirja Krause and Rik K.Wierenga561
16.1 Introduction562
16.2 General Aspects of Protein Engineering566
16.2.1 Library Creation Methods569
16.2.2 Structure-Based Library Design572
16.2.3 Optimal Libraries for Directed Evolution Methods574
16.2.4 Data-Driven Design (Semirational Design)578
16.2.5 Protein Engineering by Selection and Screening Methods579
16.3 Directed Evolution Studies with TIM-Barrel Enzymes584
16.3.1 Protein Engineering Studies of TIM-Barrel Proteins586
16.3.2 The Kemp Eliminases590
16.4 Concluding Remarks596
17 Handling the Numbers Problem in Directed Evolution&Carlos G.Acevedo-Rocha and Manfred T.Reetz613
17.1 Introduction614
17.2 Saturation Mutagenesis in Directed Evolution617
17.3 Statistical Analyses620
17.3.1 Conventional Statistics Based on the Patrick and Firth Algorithm620
17.3.2 Statistics Based on the Nov Algorithm624
17.4 How to Group and Randomize Amino Acid Positions626
17.5 Fitness Landscapes628
17.5.1 Fujiyama vs.Badlands Fitness Landscapes628
17.5.2 Fitness-Pathway Landscapes and How to Escape from Local Minima630
17.6 Conclusions and Perspectives636
18 Hints from Nature:Metagenomics in Enzyme Engineering&Esther Gabor,Birgit Heinze,and Jurgen Eck643
18.1 Metagenomics and the Ideal Enzyme644
18.2 Molecular Microdiversity647
18.3 Metagenomic Enzyme Chimera650
18.4 Outlook653
19 A Functional and Structural Assessment of Circularly Permuted Bacillus circulans Xylanase and Candida antarctica Lipase B&Stephan Reitinger and Ying Yu657
19.1 Introduction657
19.2 Naturally Occurring Circular Permutations:Selected Examples658
19.3 Circular Permutation of Bacillus circulans Xylanase661
19.4 Circular Permutation on Candida antarctica Lipase B669
19.5 Conclusion674
20 Ancestral Reconstruction of Enzymes&Satoshi Akanuma and Akihiko Yamagishi683
20.1 Introduction683
20.2 Reconstruction of an Ancestral Protein Sequence684
20.2.1 Overview684
20.2.2 Methods for Ancestral Sequence Reconstruction684
20.2.3 Early Works686
20.3 The Commonote687
20.3.1 The Last Universal Common Ancestor,the Commonote687
20.3.2 Theoretical Studies on the Environmental Temperature of the Commonote688
20.3.3 Reconstruction of an Ancestral Nucleoside Diphosphate Kinase689
20.3.4 Estimation of the Environmental Temperature of the Commonote692
20.4 Application to Designing Thermally Stable Proteins693
20.4.1 Design of Thermally Stable Proteins693
20.4.2 Case Studies to Create Thermally Stable Enzymes by Introducing Ancestral Residues as Amino Acid Substitutions694
20.4.3 Reconstruction of Thermally Stable,Ancestral DNA Gyrase Using a Small Set of Homologous Amino Acid Sequences696
20.5 Conclusion697
PART Ⅳ ENZYME SCREENING AND ANALYSIS707
21 High-Throughput Screening or Selection Methods for Evolutionary Enzyme Engineering&Shuobo Shi,Hongfang Zhang,Ee Lui Ang,and Huimin Zhao707
21.1 Introduction708
21.2 Selection710
21.2.1 Solid-Medium-Based Selection717
21.2.2 Liquid-Medium-Based Selection719
21.2.3 Display-Based Selection722
21.3 Screening724
21.3.1 Chromatography- and Mass-Spectrometry-Based Screening725
21.3.2 Solid-Medium-Based Screening726
21.3.3 Microtiter-Plate-Based Screening727
21.3.4 Yeast Two-/Three-Hybrid System729
21.3.5 FACS-Based Screening729
21.3.6 Microfluidics-Based Screening732
21.4 Conclusions and Prospects734
22 Nanoscale Enzyme Screening Technologies&Helen Webb-Thomasen and Andreas H.Kunding745
22.1 Introduction745
22.2 Approaches to Nanocompartmentalization of Enzymes746
22.2.1 Liposomes747
22.2.1.1 Addressability747
22.2.1.2 Reagent exchange749
22.2.2 Polymersomes and VirusLike Particles751
22.2.3 Water-in-Oil Emulsion Droplets752
22.2.3.1 Addressability755
22.2.3.2 Reagent exchange755
22.3 Microfabricated Chip Devices for Enzyme Compartmentalization and Screening756
22.3.1 Microfluidic-Generated Emulsion Droplets757
22.3.2 Microfabricated Arrays762
22.3.2.1 Optical fiber microarrays762
22.3.2.2 Elastomeric microarrays763
22.3.2.3 Surface tension microarrays765
22.4 Conclusion and Current Challenges767
22.5 Future Improvements769
23 Computational Enzyme Engineering:Activity Screening Using Quantum Chemistry&Martin R.Hediger777
23.1 Motivation778
23.2 Introduction779
23.3 Methods780
23.3.1 Calculation Engines780
23.3.2 Molecular Modeling782
23.3.3 Software786
23.4 Applications786
23.4.1 Overview786
23.4.2 Engineering Candida antarctica Lipase B787
23.4.3 Engineering Bacillus circulans Xylanase793
23.5 Conclusions800
24 In Silico Screening of Enzyme Variants by Molecular Dynamics Simulation&Hein J.Wijma805
24.1 Potential Applications of MD Simulations For Improving Enzymes805
24.2 Molecular Dynamics vs.Other in silico Methods809
24.3 Improving Catalytic Activity by MD Screening812
24.3.1 Transition-State Simulation812
24.3.2 High-Energy Intermediate Simulation814
24.3.3 Substrate Simulation with Near-Attack Conformations815
24.3.4 Substrate Simulation with Monitoring of H Bonds817
24.4 Predicting and Improving Binding Affiinity818
24.5 MD Screening to Improve Enzyme Stability819
24.6 Improving Correlation between MD and Experiment822
24.6.1 Force Field Inaccuracies822
24.6.2 Sampling Concerns823
24.6.3 Other Concerns824
24.7 Outlook and Further Possibilities825
25 Kinetic Stability of Variant Enzymes&Jose M.Sanchez-Ruiz835
25.1 Kinetics vs&Thermodynamics in Protein Stability835
25.2 Mutation Effects on Kinetic Stability:A Description Based on the Transition State for Irreversible Denaturation838
25.3 Kinetic Stability Linked to the Breakup of Interactions in the Transition State:Pro-dependent Proteases841
25.4 Kinetic Stability Linked to Substantially Unfolded Transition States:Thioredoxin and Phytase Enzymes842
25.5 Role of Solvation Barriers in Kinetic Stability:Lipases and Triose Phosphate Isomerases848
25.6 Concluding Remarks852
Index859